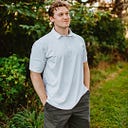

Discover more from Holodoxa
This article is part of a series on hereditary cancer syndromes and cancer genetics called Cancer Genomes. If new to the series, please go to my post “Introducing Cancer Genomes” for an explainer.
tl;dr
Although most cancer is not inherited, all cancers are genetic in origin. The normal genetic program of a cell has been disrupted, typically by spontaneous mutation, causing that cell to proliferate and evade destruction by the body’s defenses. Over the life of the budding tumor, the tumor’s genetic landscape changes at rapid pace and changes in different ways within the tumor itself. This is prime tinder for an evolutionary bonfire. Doctors can treat the cancer in any way and there will still likely be some part of the cancer that is resistant to the treatment and will eventually reseed the body with disease. Thus, treating cancer is a relentless struggle against a protean foe pushing evolutionary possibilities to the maximum. Yet, we increasingly have the tools to study and monitor this hyper-Darwinian process. This may prove to be our best hope for getting ahead of cancer evolution and thus get better at treating cancer.
The conventional triumvirate of cancer treatments — surgery, chemotherapy, and radiation — have shown a robust capacity to shrink tumors and extend patient survival. These options, though effective, often come at a terrible cost, debilitating side effects. We are moving into an era where precision treatment options can be used to complement or replace of these blunt anti-cancer tools. However, we still face the near Sisyphean task of beating a souped-up version of evolution at its own game.
Now, not all cancer is this impossible to defeat. There is a fairy clear relationship between how long the cancer has been germinating and eluding detection and how resistant it will be to treatment. In cases of early-stage disease, thorough surgical removal, where surgical oncologists and pathologists confirm that all disease has been removed, may itself suffice for sustained remission. But don’t let this scenario give you too much hope. Even the most skilled surgeons cannot guarantee lasting remission when excising a primary tumor despite no signs of invasion or metastasis. Although not abundantly evident, there are hints in surgical data that suggest the act of surgery itself might inadvertently promote the scattering of cancer cells from the primary site. The likely mechanism for this dispersal during surgery lies in the very nature of the procedure, which compromises tissue and vascular integrity near the tumor, potentially hastening the migration of cancer cells into neighboring tissues or even into the circulatory or lymphatic systems.1
Unfortunately, many cancer are diagnosed at far more advanced stages, locally advanced or metastatic disease. These clinical scenarios are immediately more complex. With invasive cancers, those where malignant cells have migrated from the original tumor site into neighboring healthy tissue, have already spread and likely evolved in ways that increase their ability to elude detection and survive therapeutic onslaughts.
My reference to the cancer’s evolution, specifically refers to the genetic diversity in the pool of cells comprising the advanced cancer. These cells have accumulated many new mutations as they’ve grown and divided. Some of these mutations may have already been selected for their ability to promote the growth and survival of the cancer. Cancer has to evade the body’s own immune system and fight for nutrients so selection pressures are already in place before medical treatments even begin. The more this goes on the greater the pool of genetic variation the cancer has to draw from. This means the cancer has more paths to become resistant to whatever treatment is thrown at it.
It gets worse too. Even when a given treatment has seemingly destroyed all advanced disease, there are likely latent malignant cells lurking in the body. These latent cells, having eluded therapy, can rapidly re-seed the body with disease, while also eluding some conventional methods of detection - whether our own body’s or the clinician’s. For instance, the relative dormancy of these resistant cancer clones, often called cancer stem cells, means they may not be detected by glucose absorption imaging.2
Before we jump into the molecular evolution of cancer in detail. Let’s return to the remainder of our anti-cancer triumvirate: cytotoxic drugs and ionizing radiation. These therapies are often given as an adjuvant to surgery in an attempt to eradicate any cancer cells lingering in or around the surgical site, as well as those that have disseminated to proximal lymphatic nodes. Although it was once presumed that the capacity for invasion and metastasis emerged later in a tumor’s development, current research indicates that such invasive cells might embark on their migration from the primary site quite early in certain cancers.
The Armamentarium
Chemo & Radiation
Chemotherapy and radiotherapy are the stalwarts of conventional cancer treatment. They work by disrupting key cellular functions by delivering supra-physiological insults. Chemotherapies disrupt cellular processes integral to rapidly dividing cells: DNA synthesis, DNA repair, and cytoskeletal architecture. Radiation bombards the DNA of cells, increasing mutations in the tissue in the treatment area. In both cases, extensive genomic injury caused by these agents hampers DNA replication, leading to cellular demise via self-destruct (apoptotic) pathways. This process can also activate the immune system to target and eliminate any cancer cells that withstood initial therapy. The rationale behind using ionizing radiation against cancer is the assumption that cancerous cells, with their compromised DNA repair capabilities, cannot mend the genetic harm inflicted during treatment. In contrast, healthy cells, with their DNA repair mechanisms intact, are able to recover from such damage. This principle is similarly applicable to DNA-targeting chemotherapeutic drugs like platinum agents.
Both treatment approaches acknowledge there will be a lot of off-target killing of healthy cells. This is where many of the well-known side effects of chemotherapy and radiation (hair loss, nausea, etc) arise from.
Precision Agents
After the completion of the human genome project and the advent of next-generation sequencing (NGS), a rapid growth in the field of precision oncology followed. Precision oncology includes molecular analysis of a patient’s tumor followed by matching of treatment with that molecular profile. In contrast to the blunt, burn-it-all-down approach of chemo- and radiation therapy, targeted agent are developed to be specific to cancer drivers that can be identified by molecular diagnostic tests. Ideally, this maximizes therapeutic benefit and minimizes off-target effects. 3
As of this writing, the most robustly developed precision paradigm exists for the treatment of non-small cell lung cancer (NSCLC). The national cancer comprehensive network (NCCN), a panel of experts, recommends that any patient with advanced NSCLC receive upfront comprehensive genomic profiling (CGP) so that their eligibility for the many approved targeted agents can be assessed. Impressively, there are already nine different specific biomarkers that have approved agents in this setting.
A subset of precision drugs includes the immunotherapy (IO) class. The most famous IO is Keytruda (Pembrolizumab), an anti-PD-1 monoclonal antibody. These drugs help refocus the body’s immune system on the cancer by disabling the cloaking mechanism the tumor deploys. These agents have proved especially potent in certain setting, i.e. “hot” or genomically unstable tumors. This has included cancers like melanoma. For instance, former President Jimmy Carter is still alive today despite a diagnosis of melanoma with brain metastasis in 2015.
The hope of outrunning cancer evolution depends on developing and implementing total precision oncology. Complete or even sufficient knowledge of the molecular drivers throughout a cancer case should reveal what can be done to stop it. Plus, we can use these precision approaches to monitor the cancer as it responds to treatment in real-time. This may enable us to get ahead of resistance or chase cancer into dead-ends of its own making. A realization of this dream won’t mean dispensing with the conventional triumvirate of cancer treatment. It will mean leveraging molecular data to guide how former one-sized-fits-all approaches can be used.
The Aces up Cancer Evolution’s Sleeves
Drug Resistance
Certain cancers rely on the continuous activity of oncogenes to progress, a phenomenon known as “oncogene addiction.” HER2 positive (HER2+) breast cancer is a prime example, where the HER2 receptor (gene name ERBB2) — a relative of the epidermal growth factor receptor (EGFR) — is excessively produced (overexpressed). HER2, pivotal in breast tissue development and maintenance, when overexpressed, can be targeted with certain anti-HER2 drugs like Herceptin (trastuzumab), a monoclonal antibody. This treatment, which inhibits the HER2 receptor, has yielded remarkable and lasting effects in some instances. Because the patent on trastuzumab has elapsed, drugmakers have iterated on the original design improving it in clever ways. For instance, there is now an antibody-drug conjugate called Enhertu (fam-trastuzumab deruxtecan) consisting of trastuzumab linked to a cytotoxic drug. This is an exciting way to combine a targeted approach with chemotherapy in a way that optimize for therapeutic benefit and cuts down on side effects.
However, advanced cancer treatments still face obstacles similar to traditional chemotherapy, such as the emergence of resistance. The intricate web of biological pathways offers multiple routes for cellular response, allowing surviving cancer cells to eventually circumvent the targeted pathway of the drug. Classically, this includes a new mutation that enables the cancer cells to neutralize the effect of a drug. Some of the resistance strategies don’t directly resist a drug’s mechanism of action, but create a backdoor for the cancer to escape through. For instance, cancer cells can adapt by reducing drug absorption or activating emergency genes that promote survival and division.
There are other ways to conceive of mechanisms of resistance. Some prefer classifying the different mechanisms into intrinsic versus extrinsic types. Intrinsic resistance involves changes within the cancer cells themselves, such as mutations that alter drug targets or cellular pathways, allowing cells to evade the effects of drugs. Extrinsic resistance arises from changes in the tumor microenvironment (TME) that bolster cancer cell survival, such as the secretion of proteins by normal cells in response to the tumor-induced inflammatory milieu, which inadvertently supports tumor growth.
Resistance is a major factor in the limited long-term success of many cancer therapies. Even with targeted biological treatments that emerged in the 1990s, like monoclonal antibodies or small molecule tyrosine kinase inhibitors, resistance is a frequent occurrence. As cancer cells evolve, mutations arise that enable some to circumvent the obstacles posed by targeted treatments. Even when early interventions show promising results, including the seeming elimination of the primary tumor or reduction of metastatic growths, resistance can still develop over time (cancer evolution). One strategy that has proven effective on this front has been combination therapies. This is when two or more drugs are given together to block both the current driver of the cancer and the likely evolved resistance pathway. One of the best examples of this approach is seen in melanomas with BRAF V600E mutations. These patients can receive dual blockades of BRAF and MEK via drug combinations like Tafinlar (dabrafenib) and Mekinist (trametinib).
Tumor Heterogeneity
Part and parcel of the phenomenon of resistance is the fact that cancer functions as a tissue. Simplistic conceptions of cancer as a population of clones have often dominated thinking the field. Simple models can be powerful, but we have learned the hard way that we can’t ignore the complexity of cancer. This complexity is referred to as “tumor heterogeneity” and simply means that there are important differences between cancer cells within a single tumor and across different metastatic lesions.
The diversity within tumors and the dynamism of tumors provide a huge survival advantage to cancer. The cancer has an enormous pools of different cellular phenotypes that it can rely on to respond to whatever threat it faces. Until recently, we had few means to asses the diverse biology of tumors. Thanks to recent innovations often on top of NGS platforms we now can peak in at the hyper-Darwinian process at work inside of tumors.4 One of the discoveries, though somewhat controversial, that has emerged from studying tumor heterogeneity is the existence of cancer stem cells (CSCs).
Cancer Stem Cells (CSCs)
CSC are cells within tumors that have the ability to self-renew, differentiate, and form tumors when transplanted into an animal. They have a “stem-like character” that the other cells of a tumor do not. Like adult stem cells, they serve as a reservoir to replenish tissue when called upon but can otherwise lie quiescent. They’re cells that follow the Teddy Roosevelt maxim, “Speak softly but carry a big stick.”
Given that chemo- and radiotherapy primarily affect actively dividing cells, they are less effective against CSCs, which are relatively dormant. They are not engaged in nutrient uptake for biosynthesis or DNA replication, and thus, they are less likely to absorb the therapeutic agents designed to disrupt cell division. The quiescent phase of CSCs can span years or even decades, shedding light on the lengthy intervals that can occur between a seeming cure and the resurgence of cancer much later. These latent CSCs are akin to ticking time bombs, possessing a more stem-like, invasive, and aggressive nature than their forebears. This characteristic also underscores why combating metastatic cancer remains one of the most formidable challenges in medicine. For years, the strategy in cancer treatment has been to poison cancer cells more than normal cells, hoping the latter can recover from the assault. However, this approach also compromises normal cells, including those vital to the immune system, which plays a crucial role in coordinating the body’s defense mechanisms. The tradeoff inherent to this treatment approach may have little utility to patients if we know CSCs will whether the attack.
The cancer stem cell theory offers a persuasive part of the rationale for cancer’s resilience. Nestled within their protective niches and coaxed into mesenchymal states by the surrounding stromal cells, cancer stem cells persist and evolve. Eventually, they may break away from the primary tumor, invade neighboring tissues, and embark on a perilous quest for a new haven.
The future of effective cancer treatment lies in finding new strategies that target the entire spectrum of cancer cells, particularly the stem cells. Selectively targeting these stem cells could circumvent complications like tumor lysis syndrome, a potentially fatal condition caused by the release of cellular contents from a mass of dying cells after treatment, leading to systemic organ damage.
The Tumor Microenvironment
There is another layer of complexity that is relevant to resistance and tumor heterogeneity, the tumor microenvironment (TME). The TME where a tumor has made its home. It is a dynamic landscape, constantly altered by chronic inflammation, hypoxia, acidosis, and high levels of reactive oxygen species (ROS), coupled with genetic instability. This environment is a mosaic of micro-niches, each with its own ROS, oxygen, nutrient, and metabolite levels, creating a breeding ground for the evolution of various cancer cell clones. The TME is not normal tissue. The cancer has remodeled to ensure it has a state-of-the-art security system, a cancer panic room or cancer fortress, if you will.
The TME begins when normal cells react to the tumor as they would to a wound and initiate protective inflammatory responses. The start to break down damaged cells and begin tissue repair. This well-intentioned response, however, often inadvertently aids in tumor progression because the tumor cells are granted access to an arsenal of biochemical and cellular weapons they can wield to advance their relentless campaign toward metastasis. This arsenal includes growth factors that fuel cell division and a suite of proteins like transcription factors that not only deter cell death but also foster an inflammatory environment conducive to tumor growth, vascular development, and the invasion of surrounding tissues. These proteins are secreted, crossing the cell membrane into the extracellular space, where they break down the barriers between cells, facilitating the spread of cancer. Thus, the non-cancerous cells within the tumor microenvironment (TME) become casualties, co-opted by the cancer cells into aiding their own demise.
Within the TME’, the interplay of cellular diversity and genetic instability/variation intensifies the evolutionary pressure on cancer cell clones. Over time, this pressure leads to the attrition of many cancer cells, leaving behind a cadre of resilient survivors. These survivors, the most formidable of the cancer cell clones, thrive under the TME’s paradoxically harsh selective pressures and hospitable conditions, outmaneuvering their counterparts for scarce resources. Paradoxically, it is the very therapies aimed at combating cancer that can inadvertently foster the progression of these tenacious cells, creating chemo-resistant pockets that may later become the cradle for disease recurrence.
Can Our Knowledge and Technology Outrun Evolution?
The hyper-Darwinian process of cancer presents the most formidable challenges to medicine: beat a revved up version of evolution at its own game. There are some advantages on our side of the ledger though. We can benefit from past research and development, the numerous observations of the cancer process at work. Every time a cancer patient is studied closely with molecular tools, we can get a better foothold to forge forward against cancer. We owe it to the cancer patients of the present and future to learn as much as possible today about the diverse molecular underpinnings of cancer and how those cancer respond to various treatment modalities.
In other words, we have the means and opportunity to kill cancer, but we need the sufficient motivation to take the risks and make the investments necessary to finish the job. Of course, it is important to realize that the killing of cancer will likely never be final. Our genome is expansive and undoubtedly host an untold number of tumorigenic pathways that already exist or can emerge. Nonetheless, if we can push cancer research and care to the point where we can confidently slam on the different breaks we already know are there and monitor responses in real-time with exquisite precision, then it is possible we can at least return meaningful about of high quality life years to patients who today have terminable disease with a near guarantee of rapid decline.5
There are some wild theories of cancer initiation and progression that concern tissue architecture and symmetry. This is not the dominant theoretical model, and there is yet to be an effective treatment derived from related observations. For a curious reader, here are a couple example of this: study and theoretical paper.
F-fluorodeoxyglucose (FDG) positron emission tomography (PET) is often used to identify metastatic lesions. Cancer cells tend to have abnormal metabolic profiles relative to healthy cells.
There is substantial variability in the efficacy and safety profiles of precision therapeutics. They’re not necessarily by default better than the one-sized-fits-all approaches to treating cancer. However, we have a growing armamentarium and body of research to demonstrate when such approaches are more effective and safer.
Here, I am referring to approaches in single-cell sequencing, spatial transcriptomics, and flow cytometry.
Another approach that will be important will be earlier and more accurate diagnosis. The practice of multi-cancer early detection (MCED) is emerging and may significantly lower the number of patients who are diagnosed with later stage disease. There are known limitations to cancer screening, but the precision of NGS may help liberate us from such problems.
Subscribe to Holodoxa
A study of the total human condition. I examine ideas from science, literature, technology, politics, and more. Most posts will consist of book reviews or short essays.
Custom vaccines are exciting: https://jakeseliger.com/2024/04/12/moderna-mrna-4157-v90-news-for-head-and-neck-cancer-patients-like-me/
Nice review of the pristine molecular biology of cancer genomics and the hope of Next Gen precision oncology. Despite the biologic complexity of cancer growth, it is not surprisingly that lay folks believe the “cure for cancer” is hidden because of profit motives as they are rightfully suspicious of Big Pharma and the big business of cancer chemotherapy given the war on cheap, generic repurposed drugs, the known “kick-backs” to prescribers with the financial “margins” on expensive and toxic non-targeted prescribed chemotherapy as well as the well documented regulatory capture of the FDA, the industry funded research manipulation of data and the lack of attention to inexpensive cancer reducing therapies-vitamin D, avoidance of tobacco/ETOH/Diabesity.